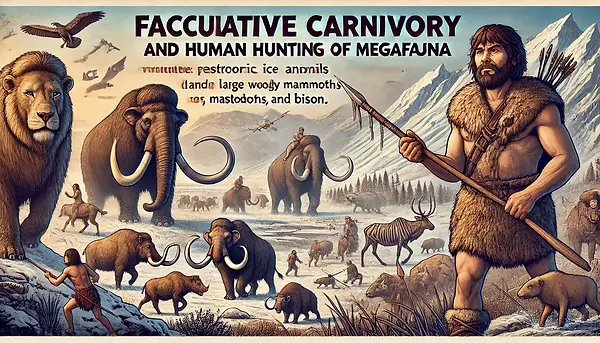
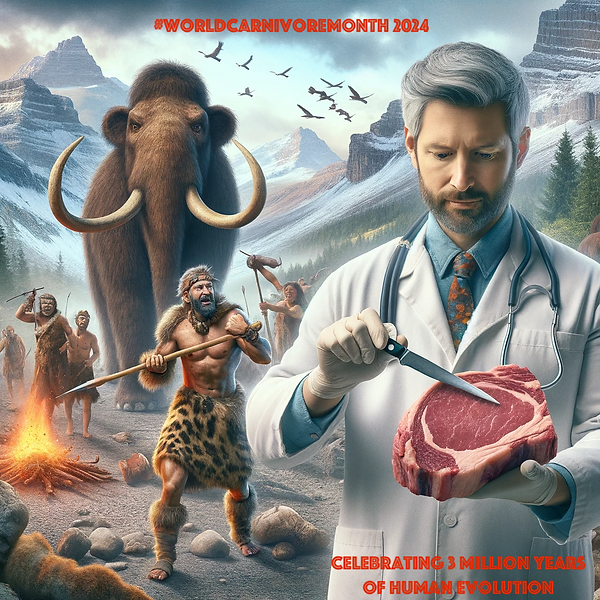
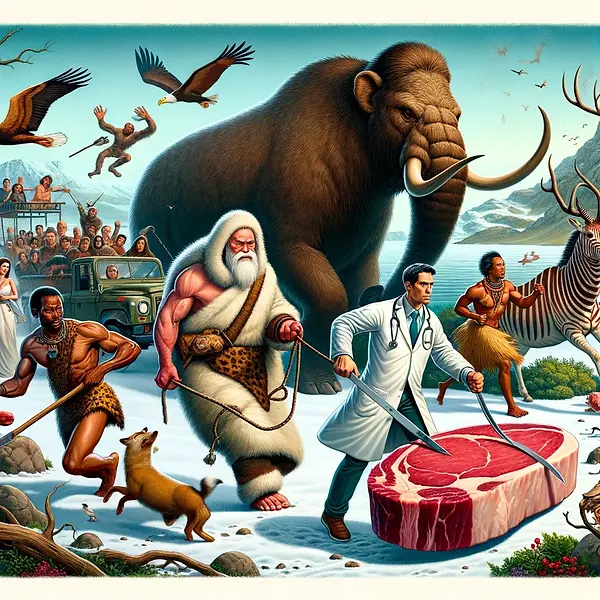
Title:
Reframing Nutritional Microbiota Studies To Reflect an Inherent Metabolic Flexibility of the Human Gut: a Narrative Review Focusing on High-Fat Diets
Abstract:
There is a broad consensus in nutritional-microbiota research that high-fat (HF) diets are harmful to human health, at least in part through their modulation of the gut microbiota. However, various studies also support the inherent flexibility of the human gut and our microbiota’s ability to adapt to a variety of food sources, suggesting a more nuanced picture. In this article, we first discuss some problems facing basic translational research and provide a different framework for thinking about diet and gut health in terms of metabolic flexibility. We then offer evidence that well-formulated HF diets, such as ketogenic diets, may provide healthful alternative fuel sources for the human gut. We place this in the context of cancer research, where this concern over HF diets is also expressed, and consider various potential objections concerning the effects of lipopolysaccharides, trimethylamine-N-oxide, and secondary bile acids on human gut health. We end by providing some general suggestions for how to improve research and clinical practice with respect to the gut microbiota when considering the framework of metabolic flexibility.
Details
https://pmc.ncbi.nlm.nih.gov/articles/PMC8092254/
REFRAMING A HEALTHY GUT IN TERMS OF EVOLVED FLEXIBILITY
New technologies and greater interest in gut health in recent years have dramatically increased our understanding of gut microbes. Nevertheless, we are still unable to define a “healthy” gut microbiota (22, 25). On average, any two individuals share only about a third of their gut microbiota, with the other two-thirds varying depending on genetics, geographical location, history of antibiotic and medication use, mode of delivery at birth, diet, and other undetermined factors (43, 44). It is even possible that two otherwise-healthy individuals can show no overlap in microbiota composition (44). Thus, outside clear instances of dysbiosis, we have insufficient information to say that one individual’s “two-thirds” is any better than another’s.While it is generally believed that diversity and community stability are key components of a healthy gut ecosystem, even these can sometimes be associated with diseased states (45, 46). Some of the keystone microbes commonly considered crucial for gut health, such as Bifidobacterium, are completely absent from the guts of traditional cultures, like the Hadza, who are otherwise virtually free of chronic disease (47). Gut health and dysbiosis thus remain vague and sometimes contested concepts (25, 48, 49); if there is a healthy “core” microbiota, it may be at the level of microbial functions, not species (23, 43). Part of this relative lack of insight may result from technologies such as 16S rRNA sequencing, which do not provide accurate information beyond the genus level and provide little insight into microbial functions (50). While there is hope that higher-resolution technologies (e.g., metagenomics, metabolomics), larger data sets, and advanced computing techniques will bring us closer to defining a healthy microbiota, many researchers call for moving away from cataloguing species and toward an approach that considers the intricate nature of microbiota-host interactions (22, 51).While technological advances are eagerly awaited, some initial clarity might come from placing the human microbiota in its evolutionary context. Our relationship with our gut microbes is the product of thousands of generations of close coevolution (52, 53). The environments in which we evolved also required regular adaptation to changing conditions. Our ancestors may not always have had steady access to food and would likely have undergone occasional bouts of significant deprivation when food was scarce (54, 55). Similarly, diets changed seasonally and geographically, as is reflected by the seasonal changes in the guts of traditional populations, like the Hadza (56), or in the specific adaptations in cultures known to eat relatively few plant foods, such as the Inuit (57, 58). This variability can be explained in terms of metabolic flexibility (19), which is the evolved ability to shift our metabolism to changes in dietary intake: to burn and use carbohydrates when they are plentiful and to turn dietary fat or stored body fat into ketones for energy when food or carbohydrates are scarce. Consequently, it seems likely that our guts also exhibit the flexibility to adapt to changing food sources rather than suffer significant gut dysfunction whenever fiber is absent.In line with this evolutionary perspective on the compositional and functional adaptability of our gut and its microbiota, David et al. write (18),
Our findings that the human gut microbiome can rapidly switch between herbivorous and carnivorous functional profiles may reflect past selective pressures during human evolution. Consumption of animal foods by our ancestors was likely volatile, depending on season and stochastic foraging success, with readily available plant foods offering a fallback source of calories and nutrients. Microbial communities that could quickly, and appropriately, shift their functional repertoire in response to diet change would have subsequently enhanced human dietary flexibility.In other words, a “healthy” gut microbiota adapts to a wide range of food sources and does not necessarily become more or less pathogenic depending on the amount of carbohydrate or fat in the diet. Moreover, while short-term dietary changes tend to produce significant changes in the gut microbiota (18), long-term studies suggest a relative resilience of the microbiota to shifts in diet (59). Due to the aforementioned factors shaping gut microbiota (43, 44), we should consider whether a dietary change must produce significant physiological changes in the host before a new microbial stability is achieved, with diet-induced fluctuations merely an expression of the gut’s ability to adapt to ensure optimal function. If human guts are inherently metabolically flexible, short-term diet-induced changes in microbiota composition could be considered a potential hallmark of gut health (60). We should thus determine whether the short- and long-term taxonomic changes resulting from this metabolic flexibility are predictive of overall health outcomes and how/whether the microbiota drives those outcomes (61–63).
EVIDENCE FOR HIGH-FAT KETOGENIC DIETS—CONSIDERING ALTERNATIVE PATHWAYS
Gut bacteria metabolize complex carbohydrates to produce short-chain fatty acids (SCFAs), like acetate, propionate, and butyrate, with the last being the preferred fuel source for gut epithelial cells. Published estimates suggest that butyrate provides about 70% of colonic epithelial cell energy requirements (64), with a regular supply of butyrate required to maintain gut barrier function. What remains to be seen is how different diets modulate SCFA production and whether this results in different downstream health effects.
Animal-based diets.
The work of David et al. (18) has been instrumental in highlighting how quickly and reliably the human gut microbiota adapts to dietary changes. What is unclear is whether this study should be used to support the avoidance of diets high in fat or protein. Ten healthy human volunteers were placed on a short-term plant-based diet (PBD) consisting of 300 g of carbohydrate per day from cereal, vegetables, rice, lentils, and fruit or on an animal-based diet (ABD) consisting of less than 3 g of carbohydrates per day, with 30% of calories from protein and 70% of calories from fat from eggs, meat, and cheese. Subjects on the ABD group were confirmed to be in ketosis by day 2 of the diet, with distinct gut microbial communities emerging in both diet groups within 3 days.The most interesting and perhaps contentious finding was that there was no significant change in alpha-diversity in either group (18). Those on the ABD saw an increase in the relative abundance of bile-tolerant microorganisms, like Bilophila, Alistipes, and Bacteroides spp., and a decrease in the relative abundance of microbes known to metabolize complex dietary plant fibers, such as Roseburia, Eubacterium, and Ruminococcus spp. While often cited as evidence that an ABD is harmful, this is far from conclusive. The PBD, despite being supposedly uniquely capable of producing butyrate from microbiota-accessible carbohydrate metabolism, produced only slightly more butyrate than did the ABD, with the ABD also resulting in significantly greater production of isovalerate and isobutyrate (Fig. 1) (18). Isobutyrate has been shown to activate many of the same receptors as butyrate (see “Considering alternative pathways” below), weakening the notion that PBDs are significantly “better” for the gut due to butyrate/SCFA production.
Mucus barrier.
Another recent study by Ang et al. confirmed that a ketogenic diet (KD) can alter the structure and function of the gut microbiota (65). In humans, among the most significant changes of the fecal microbiota following a KD was a dramatic reduction in the abundance of several Bifidobacterium species. In controlled-feeding studies of mice, the researchers found that KDs had a unique impact on the gut microbiota relative to conventional HF diets, with the abundance of Bifidobacterium organisms decreasing with increasing carbohydrate restriction. Further experiments found that both a KD or ketone ester supplementation increased beta-hydroxybutyrate (βHB) in the lumen of the gut and in colon tissue (65), with ketone bodies directly inhibiting the growth of Bifidobacterium. Ketosis was also associated with a reduction in small intestinal Th17 cells, which help maintain the gut mucosal barrier and contribute to pathogen clearance at mucosal surfaces. However, Th17 cells have also been implicated in autoimmune and inflammatory disorders (66).
Next, Ang et al. (65) sought to determine whether the change in Th17 cells was dependent on the ketone-induced changes in the microbiota. Mice that received a fecal transplant of the ketone-fed microbiota from human donors had significantly fewer intestinal Th17 cells. Contrary to previous findings that mice fed fiber-free diets had a significant breakdown of the colonic mucus layer (67, 68), Ang et al. write, “A ketogenic diet maintains a robust mucus layer despite the lack of fermentable carbohydrates” (65). The KD maintained not only the thickness of the mucus layer but also the expression of Muc2, the primary constituent of gut mucus. Nutritional ketosis might actually support the gut mucus layer.
Considering alternative pathways.
Multiple strands of evidence question an assumption about “normal” metabolic pathways in the gut. Alongside the SCFAs mentioned above, there are several other molecules that can serve as sources of fuel for gut epithelial cells. The very idea of a “preferred” fuel source may be skewed from studying people (and rodents) who eat a large amount of microbiota-accessible carbohydrates. In other words, while butyrate production may be reduced on a KD, other molecules can potentially take butyrate’s place to help maintain gut barrier function.
This shift to an alternative pathway is what we might expect from the perspective of metabolic flexibility, where we see a potential analogy between the butyrate-gut connection and glucose-brain connection. While glucose is a necessary fuel for the brain, we have known for some time that in the (relative) absence of carbohydrates, the body will shift its metabolism from glucose to fatty acids, producing ketone bodies, such as βHB, to support brain metabolism (73). This kind of fatty acid-based metabolism appears to have numerous neurological benefits and may be a “preferred” fuel for the brain during both development (74) and aging (75). Similarly, while researchers repeatedly stress that gut epithelial cells are uniquely fueled by the butyrate produced by our resident microbiota after consuming “microbiota-accessible carbohydrates” (17), here too the body is flexible, with βHB also being capable of supporting energy requirements in the gut.
In fact, there are at least four molecules that can replace butyrate: isobutyrate, acetoacetate, βHB, and bile-derived acylcarnitines (Fig. 2). Isobutyrate is a metabolite of protein fermentation that is typically produced at lower levels than butyrate. When butyrate is less abundant, isobutyrate can be absorbed from the gut lumen by gut epithelial cells and metabolized for energy (76). Fecal isobutyrate was found to be elevated in humans consuming a KD (18). Moreover, isobutyrate can stimulate the same receptors as butyrate in the gut (GPR41, GPR43, and GPR109a) to influence mucus secretion, antimicrobial peptide release, and immune regulation (77), and while isobutyrate may be produced at lower levels on a moderately high-protein diet than butyrate would be produced on a high-carbohydrate diet (18), isobutyrate appears to be a more potent stimulator of butyrate receptor GPR41 (FFAR3) than butyrate itself (78). In other words, what isobutyrate lacks in concentration relative to butyrate, it may make up for in potency. Relatedly, this may provide a reason not to confuse high-protein and refined HF diets, since the most abundant end products of protein fermentation or catabolism are SCFAs, such as isobutyrate (79). As suggested by David et al., it might be the overall context in which protein fermentation occurs that is important to downstream health outcomes rather than the protein fermentation itself.
Like butyrate, βHB can also stimulate GPR109a, reducing intestinal inflammation (80, 81). Most notably, however, both βHB and its related ketone body acetoacetate are intermediates in the pathway for butyrate metabolism; when butyrate is taken up by gut epithelial cells, it is converted into βHB first and then acetoacetate before being broken down further for energy (Fig. 2) (82). Gut epithelial cells express the monocarboxylate transporter MCT1, a primary ketone body transporter on the basolateral surface (83), and several papers suggest that gut epithelial cells are capable of utilizing ketone bodies from the vascular bed (84, 85). As gastrointestinal inflammation and mucosal damage can impair butyrate uptake from the intestinal lumen (86, 87), circulating ketones may provide a potential therapeutic option in certain patients with gastrointestinal disease.
To our knowledge, no studies have assessed the effects of ketones or a KD on gut barrier function. However, a recent study found that ketone body signaling regulates the normal function of intestinal stem cells (ISC) and their ability to respond to injury (88). In this study, β-hydroxy β-methylbutyryl-coenzyme A (HMG-CoA) synthase 2 (HMGCS2), a rate-limiting step in ketone production, was enriched in small intestinal stem cells. Ablating the Hmgcs2 gene in mice diminished βHB levels in the crypts, compromising ISC function and regeneration of the gut epithelium after injury. Exogenous βHB rescued ISC function and partially restored intestinal regeneration. A KD also increased HMGCS2 expression and ISC number, function, and postinjury regeneration. In contrast, a glucose-supplemented diet suppressed ISC ketogenesis and skewed the differentiation of ISC toward goblet and Paneth cells. Notably, once stem cells had differentiated into mature epithelial cells and migrated out of the crypt, they expressed very little HMGCS2. This suggests that mature epithelial cells do not possess the ability to generate large amounts of ketones through the classical ketogenic pathway (via condensation of two molecules of acetyl-CoA), though we know that they have the ability to utilize ketones.
Thus, it follows that if (i) a KD produces high levels of ketones in mature intestinal epithelial cells (as Ang et al. [65] found) and (ii) these are not being generated in mature epithelial cells (as is suggested by the lack of HMGCS2 in the work of Chen et al. [88]), then the ketones are almost certainly coming from circulation. Along these lines, the authors write, “Because exogenous ketones rectify Hmgcs2 loss in vitro and in vivo, liver or other nonintestinal sources of ketones may substitute or supplement ISC-generated ketones in KTD-mediated regeneration, where circulating ketone levels are highly elevated” (88).
Lastly, it has recently been shown that not only can colonic epithelial cells oxidize both short- and long-chain fatty acids but also, through mitochondrial metabolism, these cells can oxidize medium- and long-chain acyl-carnitines that are delivered from biliary secretions (89). This has clinical relevance in that a reduction in these metabolites may contribute to colonic inflammation. Importantly, this provides yet another host-derived energy source for the epithelium during low-carbohydrate diets to complement the three discussed above. We will return to this below since bile acid secretion is a common concern with HF diets.
To conclude, we suggest that a more nuanced picture of how HF diets impact gut and overall health is required, with particular attention being paid to therapeutic KDs. By considering the alternative pathways by which ketones and KDs can influence gut function, we can move toward a more evolutionarily consistent picture of human gut variability. Nevertheless, research needs to clarify whether the benefits of lower carbohydrate or KDs come directly from increasing βHB, reducing inflammation, modifying insulin and glucose metabolism, reducing caloric intake, altering the gut microbiota, or other undetermined factors.
Hypothesis:
We then offer evidence that well-formulated HF diets, such as ketogenic diets, may provide healthful alternative fuel sources for the human gut.

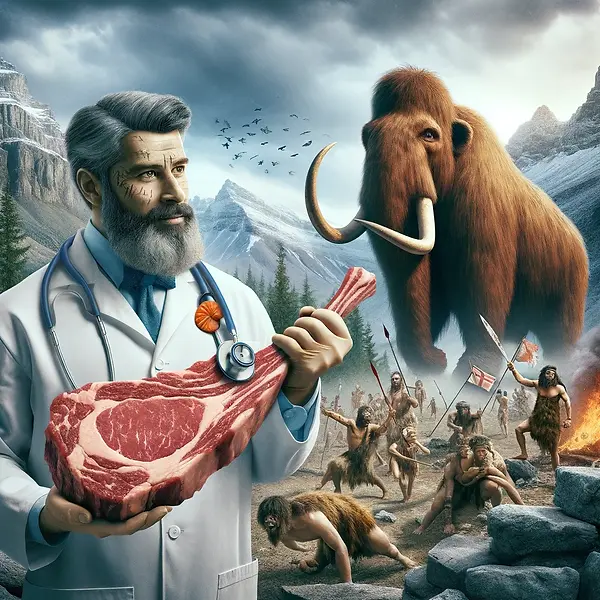
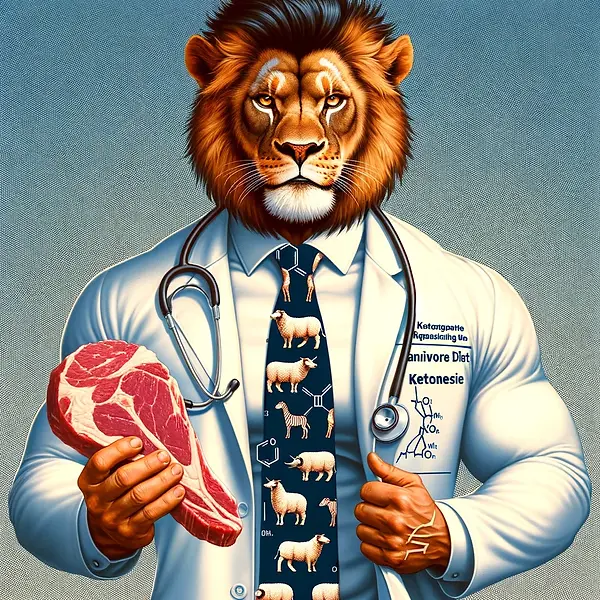